Creating
a sea change.
In the 20th
century, we extended our reach up and out to the birth of
time 15 billion years past -- and in and down to the frozen
motion of molecules. But the Hubble space telescope and scanning
electron microscopes are merely tools to let us see. What
we really want to do is visit. Just as we are pushing up and
out of the gravity well with spaceflight, we are also pushing
in and down to the bottoms of our oceans.
|
Song.
Ye
gentlemen of England
That live at home at ease,
Ah! little do you think upon
The dangers of the seas.
Martyn Parker
|
|
Octopus's
Garden
I
want to be
under the sea
in an octopus's garden
with you.
Lennon
and McCartney
|
|
Photos
courtesy: Nova "Abyss" (tubeworms),
and MBARI (squid) |
|
We already live on Waterworld: oceans cover over seventy percent
of the Earth's surface. With scuba gear, divers routinely swim to
depths of 300 feet. But everything below that is considered "deep
sea," and deep sea comprises 60% of our oceans. Here, then, is both
the opportunity and the risk: the ocean depths offer more daunting
technical challenges than deep space.
But, you think, the
vacuum! temperature fluctuations of several hundred degrees! radiation!
Space vehicles must tolerate sunside temperatures of 250 F in tandem
with lows of 250 F, and shield their contents against both
radiation and space debris. Yet deep ocean vehicles must tolerate
a similar temperature variance -- 35 F in the coldest depths to
750 F near hydrothermal vents -- as well as protect against one
of the most corrosive substances on Earth: saltwater. Where astronauts
need only suit up for a one atmosphere difference in pressure, divers
endure a one atmosphere increase in pressure for every 33 feet (10
meters) they descend.
Furthermore, as Marcia
McNutt, CEO of MBARI (Monterey Bay
Aquarium Research Institute), reminds us, "solar panels are
capable of powering most interplanetary voyages, but sunlight does
not penetrate beyond the uppermost regions of the ocean. Space is
virtually transparent to the transmission of electromagnetic energy,
while the oceans are opaque to [it]." Consequently, many deepsea
exploration devices are tethered to their launch platforms. Imagine
this limitation in space. (graphic of space shuttle w/ extension
cord?)
Given these constraints, why bother? Scientists would answer, for
data: what is the largest animal on the planet, and what is its
life cycle? (The blue whale also, at 188 dB, the loudest
animal on earth.) How might plankton and algae offset rising carbon
dioxide levels in our atmosphere? (By absorbing excess.) What role
do coral reefs play in maintaining viable fish stocks? (Approximately
10% of fish caught annually for food live in reefs.) Investors would
answer, for profit: minerals on the ocean floor and dissolved in
sea water itself; biochemicals indigenous to marine plants and animals;
new food sources. And archaeologists, anthropologists, and historians
would answer, for the ancient artefacts and the stories: of sunken
cities, of lost ships, of old wars.
|
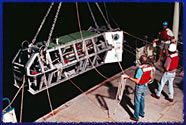 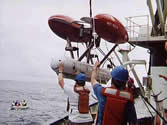 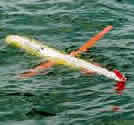
|
But let us go and make
our visit: how do we get there? Basically, we have three choices.
We can visit the ocean depths virtually, piggy-backing on the senses
of aquatic robots and remotely operated exploration tools. Or we
can build vessels that withstand the temperatures, the saltwater,
and the pressures, and visit the sea depths in the nautilus shell
of our own inventiveness. On the horizon, emerging technologies
might one day enable us to build "sea suits," and explore in conditions
more nearly skin to sea (see "Biomimetics," linked
at tubeworms and squid, above).
We extend our virtual
reach beneath the waves with three kinds of tools. Using cables,
we lower sensor arrays from ships, as the oceanographer Robert Ballard
did to explore the Titanic with the sensor sled Argo. We build undersea
robots, like Japan's Aqua Explorer 2000, or Woods Hole's ABE (Autonomous
Benthic Explorer). Or we deploy sensor stations on
the ocean floor, like the GEOSTAR station of the Mediterranean "Biodeep"
project. Economics and durability both support continued use of
towed equipment arrays, but microminiaturization, chip design, new
materials research, and biomimetic robotics (see sidebar)
point to autonomous undersea vehicles (AUVs) and robots as key marine
tools of tomorrow.
In essence, we are designing
the next benthic life-form: it will hunt anomalies, taste its surroundings,
excrete data, and return to us periodically to ingest energy for
continued existence. It may not even need batteries, using heat
engines to generate energy from ocean temperature differentials,
like the new Slocum Glider. For the sake of efficient propulsion,
this silicon seabeast will mimic its organic neighbors in design.
Draper Laboratory's newest
autonomous ocean robot is modeled after a yellowfin tuna. Simpler
additions to our technological aquarium will drift along passively
collecting data: tiny, free-floating robots will include chemical
sensors on their microchips and buckytubes to filter particulates
for analysis. These zooplankton mimics will trade and pool data
among themselves and store observations until sucked up by a larger
robofish for collection.
All this robotic sea
life will function within an ecosystem of instrumentation rather
than kelp, an ecosystem emerging from initiatives like the Neptune
project. An underwater observatory stretching from Vancouver
Island to northern California, the Neptune project will feature
2,000-miles of fiber optic cable, a host of free-roaming AUVs, and
high-definition television cameras. As of 2006, your video wallpaper
could display live feed from the deep ocean (of course, by 2015
the opposite wall may feature live feed from Mars).
|
|
In 1953 Auguste Piccard
and his son Jacques dove nearly two miles into the ocean depths
in the bathyscaph Trieste. Alvin, which currently holds the highest
use record, can only dive to about 3,000 meters. The newest 3-passenger
exploration submersible, Japan's Shinkai 6500, is sleeker, more
maneuverable, safer, and can reach depths of 6,500 meters. But even
these submersibles use twentieth century technology. Graham Hawkes,
a private entrepreneur and inventor, believes that "closer and endlessly
richer in life, resources and mysteries it is earth's oceans
not space that will fuel man's future into the next
century." His response to this imperative is the world's first underwater
"aircraft," Deep Flight. A single-person submersible, the pilot
plunges into the deep in a prone position. Inverted wings on the
craft create "negative" lift, pulling the sub into the ocean depths
without need for ballast. This enables a fast descent, optimizing
"bottom time" for exploration purposes. Deep Flight II will be proven
to over 12,000 meters with an "Ocean Everest" expedition to the
Marianas Trench.
Deep Flight's smaller
cousins will basically be atmospheric dive suits with attitude.
Atmospheric dive suits (ADS) are by definition jointed, human-shaped,
single-person submersibles which can descend to almost 760 meters
while maintaining internal pressure close to one atmosphere. This
eliminates the need for compression or decompression schedules,
but requires a suit so heavy the wearer moves it using built-in
propulsion. The next generation of ADS will easily reach depths
of 1,000 meters, include directional thrusters for increased maneuverability,
and carry enhanced sensor, data, and communication arrays onboard.
But the Exosuit, designed by Dr. Phil Nuytten, represents the most
promising future for ADS a lightweight, non-tethered dive
suit so flexible that divers will be able to swim wearing it.
|
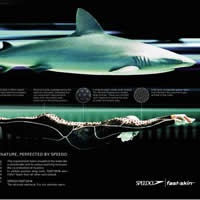 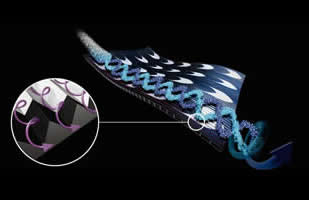 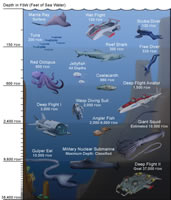 |
Scuba gear and wetsuits
come as close to swimming like fish as humans can get. New materials
developments may allow us literally to swim like fish or
like sharks and dolphins (see "Biomimetics," above).
But access to the ocean depths requires more than streamlining the
hydrodynamically clumsy human form. It means addressing the dangers
inherent in being an air-breathing mammal in a high-pressure environment.
One potential answer would be a fluid breathing apparatus, like
that spotlighted in the movie "Abyss." Replacing the air in our
lungs with a highly oxygenated fluid would sidestep the decompression
difficulties arising from breathing gases under pressure. Such a
liquid perflubron (perfluorooctyl bromide)
is currently undergoing clinical trials for therapeutic use with
patients suffering respiratory failure from infection, burns, toxic
substances, or premature birth. Significant technical problems
circulating the liquid easily through the lungs, eliminating the
carbon dioxide that is exhaled, draining the lungs in the transition
back to breathing air mean this technology will take a decade
or more to develop. But its development will allow humans much greater
freedom of the depths.
In the very long term,
beyond the scope of this book, we may know enough not only about
ourselves, but also about our marine neighbors -- dolphins, orcas,
blue whales -- to understand how they survive plummeting to great
depths and rising again to the sun and the sky, in one breath. We
may then apply that understanding to redesigning our own physiognomy.
And so, in some far future, we may need to paraphrase Shakespeare
to visualize our childrens' lives:
Full fathom five our daughters dive,
and all their bones of coral* made;
deep ocean pearls delight their eyes,
and nothing of them doth fade
but celebrates a sea-change
into something rich and strange.
*The porous, limestone skeletons of coral are being
studied for use as bone grafts.
|
|
|